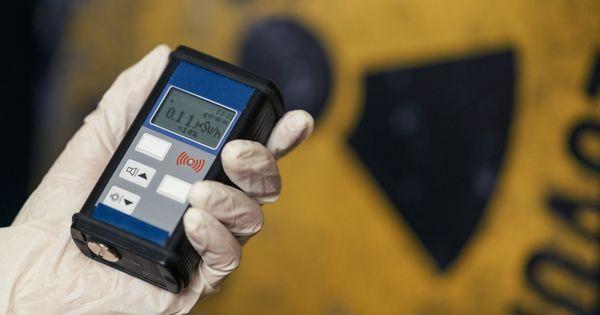
A scintillation counter is a fast and efficient solution for detecting and measuring radiation. Scintillation counters revolutionized how researchers, doctors, and other professionals detect radiation. As a result, these instruments play a key role in various fields, including cancer research, radiation surveys, nuclear plant safety protocols, and much more.
All in all, scintillation counters are remarkable instruments with a versatile range of applications. Learn more about what scintillation counters are and what they’re used for with this overview.
An Introduction to Scintillation Counters
A scintillation counter, also known as a scintillation detector, is an instrument that uses a scintillator and a photodetector to measure ionizing radiation. The scintillator responds to incident radiation by emitting photons. Then the photodetector—often a photomultiplier tube—converts those photons into an electrical signal that the instrument can process to display results.
Through this process, the scintillation counter can detect gamma rays or the presence of a particle, measure energy loss or gain, or measure radiation in a gaseous, liquid, or solid scintillating medium.
Main Components of a Scintillation Counter
A scintillation detector consists of two main components: the scintillator and the photodetector. These pieces work together to react to radiation and turn that reaction into a readable signal.
The first component is the scintillator. Generally speaking, scintillation refers to a flash of light or the process of emitting flashes of light. In this context, the scintillator produces photons—or flashes of light—as a reaction to a particle passing through the instrument. This particle might be an electron, an alpha particle, an ion, or a high-energy photon.
Attached to the scintillator is a photodetector. This photodetector is usually a photomultiplier tube (PMT), but it can also be a photodiode or a charged coupled device. The PMT converts the photon from the scintillator into an electrical signal that a pulse discriminator or digital counter can then read.
How Do Scintillation Detectors Work?
Scintillation detectors use a relatively straightforward process to detect radiation: the scintillator reacts to radiation by producing flashes of light at different intensities, and the photodetector translates those lights into an electrical signal.
The intensity of the light flashes is always proportional to the energy of the radiation. This is essential because it allows operators to precisely measure the intensity of gamma radiation and identify gamma-emitting isotopes.
To understand what scintillation counters are and what they’re used for, you must first take a deeper look at the instrument’s components and processes. The main stages of the scintillation counting process are as follows:
Radiation Moves Through the Scintillator
Scintillation counter operation begins when the radiation enters the scintillator. The scintillator material reacts to the radiation, exciting the electrons within the scintillator.
This occurs in one of two ways. When the radiation particle gets charged, it ionizes atoms along its path and creates a track of charged particles in its wake. For uncharged particles—as is the case with gamma rays—the scintillator uses the photoelectric effect, Compton scattering, or pair production to convert the energy into an energetic electron.
Either way, the resulting excited atoms then must de-excite. This de-excitement leads to the generation of low-energy photons in the visible or near-visible light range. The number of photons indicates the amount of energy the initial ionizing particle created.
Moving Through the Photomultiplier Tube
The photons leave the scintillator and hit the photocathode of the photomultiplier tube or other photodetector. The photodetector then produces a maximum of one photoelectron per photon. A voltage potential accelerates the electrons as they move through the photodetector.
When the electrons strike the first dynode, they have enough energy to release additional electrons. These secondary electrons strike the second dynode and release even more electrons. This amplification process continues through each subsequent dynode.
Reading the Results
At the final dynode, there are enough electrons to produce a detectable pulse. Each pulse correlates to one of the original photons the scintillator generated. As a result, these pulses tell operators what they need to know about the original radiation and its intensity.
Organic vs. Inorganic Scintillators
In nuclear and particle physics, most scintillation counters use one of two main types of scintillators: organic and inorganic.
Organic scintillators, also known as plastic scintillators, use organic materials to create visible light photons in response to a charged particle. These organic materials generate fluorescence in response to the energy levels of a single molecule. This fluorescence is observable as a vapor, liquid, or solid.
In inorganic scintillators, also known as crystalline scintillators, the process is somewhat different. Inorganic scintillators use crystals made from high-temperature furnaces. Examples of these types of crystals include thallium-doped sodium iodide (NaI(TI)), cesium iodide (CsI), zinc sulfide (ZnS), sodium iodide (NaI), and lithium iodide (LiI). The inorganic crystals cause a slower form of scintillation that allows them to work with higher count rates. Inorganic scintillators are highly effective for detecting gamma rays.
Advantages of Scintillation Counters
Scintillation counters offer a range of advantages that make them ideal for many applications. Efficiency is one of the main benefits. Scintillation counters can detect radiation quickly and accurately. A high level of precision and impressive counting rates deliver dependable results for operators.
Another advantage is that manufacturing scintillation counters is inexpensive, so they are cost-effective and efficient. Finally, scintillation counters can measure the intensity and energy of incident radiation, making them an extremely versatile tool.
Applications of Scintillation Counters
Scintillation counters take many forms and can fulfill a wide variety of needs. Handheld radiation survey meters, scintillation cameras, and vehicle-mounted counters are just a few examples of the reach. Some of the most common applications of scintillation counters include:
- Detecting radioactive pollution for personal or environmental purposes
- Nuclear plant safety inspections
- Medical imaging
- Rapid response damage analysis in emergencies caused by radioactive waste
- Observing protein interaction and detection
- Academic research
Additionally, scintillation detectors offer many applications in the medical field, including cancer research, cellular research, and screening technologies like in vivo and ELISA technologies. Scintillation counters also play a role in the development of pharmaceuticals, where they aid in the detection of radiolabeled compounds such as carbon-14.
Scintillation detectors are a crucial part of cGMP quality control during the development and manufacturing of pharmaceuticals. Moravek’s quality control and analytical laboratory services include two qualified scintillation counters. Learn more about our cGMP quality control services and how we deliver safe, healthy, and compliant results for your company when you contact Moravek today.
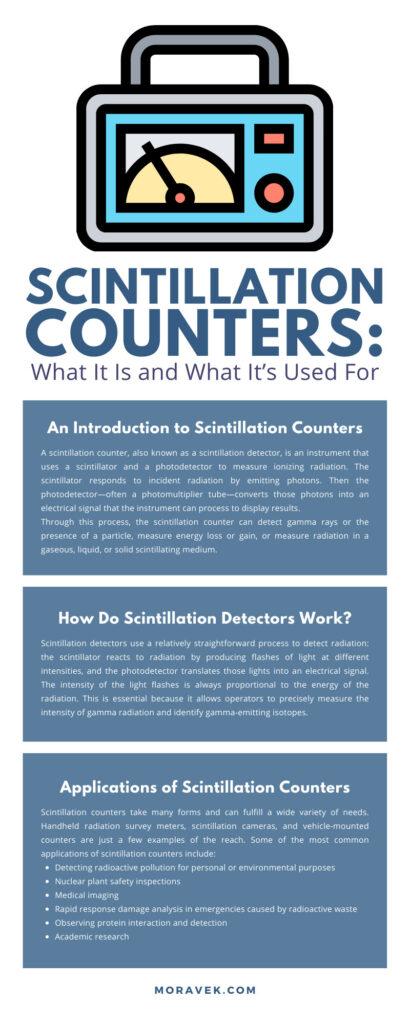